Abstract
This research paper examines the intricacies of problem-solving (PS) in organic chemistry (OC), exploring the internal and external factors that influence students’ success in this demanding discipline. Data was collected through a questionnaire administered to 128 City College of New York students during Spring and Fall semesters of 2022. The study revealed that students face challenges learning OC, which include memorization, understanding underlying concepts, and effective PS. Notably, there is a gap between students’ professed beliefs about effective PS strategies and their actual practices. While students acknowledge the importance of techniques such as annotation, trial and error, and questioning, they inconsistently apply these strategies in practice. Annotation emerged as a valuable tool, enabling students to externalize complex thought processes and reduce reliance on memorization. Trial and error, coupled with exposure to expert PS techniques, proved effective. The role of confidence in PS was highlighted, and the need to balance memorization of reactions and rules such as naming with a profound understanding of OC concepts was emphasized. Resonance as a justification for answers underscored the importance of understanding and applying principles rather than relying solely on memorization. The data highlights the importance of aligning beliefs with actions in the learning process and the need for a balanced approach that combines knowledge with effective PS techniques. The findings offer valuable implications for educators and students seeking to enhance their performance in OC.
License
This is an open access article distributed under the Creative Commons Attribution License which permits unrestricted use, distribution, and reproduction in any medium, provided the original work is properly cited.
Article Type: Research Article
INTERDISCIP J ENV SCI ED, Volume 20, Issue 2, 2024, Article No: e2407
https://doi.org/10.29333/ijese/14421
Publication date: 01 Apr 2024
Online publication date: 27 Mar 2024
Article Views: 1916
Article Downloads: 1376
Open Access HTML Content Download XML References How to cite this articleHTML Content
INTRODUCTION
For a significant portion of a student’s academic career, there is a major reliance on a cycle of memorization and regurgitation. This is particularly the case in many general courses, where students tend to memorize material because the explanation is often considered “beyond the scope of the course.” Consequently, students resort to employing their lower-order cognitive skills (LOCS) to attain academic success in these courses (Zoller & Pushkin, 2007).
However, while this memorization-heavy approach might be sufficient in general courses like biology or history, it proves inadequate in advanced courses such as organic chemistry (OC). The course requires that students employ a different set of skills altogether, including facts, logic, and reasoning. OC, on the contrary, requires a deeper understanding (Anim-Eduful & Adu-Gyamfi, 2022a, 2023) of material and employment of a higher order cognitive skills–specifically, those associated with “question-asking, critical thinking (CT), system thinking, decision making, and problem-solving (PS), as opposed to “traditional” algorithmic-based LOCS” (Zoller, 1993).
To comprehend the disparity between these contrasting learning strategies, it is crucial to define and understand PS. PS, as the name is indicative of, is a mental process that the brain engages in when a solution or answer is not immediately evident (Wood, 2006). Various models have been proposed to explain the mechanics of PS. One widely accepted model, proposed by Polya in 1946, divides PS into four distinct steps: understanding the problem and what the question is asking, and the information needed to be utilized to solve the problem, devising a plan, which include choosing an appropriate method to solve the problem, implementing the plan by applying that method to solve the problem, and reflection by double checking the answer verify that it is reasonable. The reflection phase involves the identification, prioritization, and selection of alternatives for a solution (Bodner, 2003). Notably, this PS method is universally applicable and becomes a subconscious, natural part of our thinking, seamlessly extending into the realm of OC.
While Polya’s model highlights the structured approach to PS, previous studies demonstrate that PS does not adhere to a fixed number of steps. A more holistic approach suggests that, to solve a problem, the brain picks out relevant information from the problem and, in a sense, “rewrites the problem” to interpret the goal (Bodner & McMillen, 1986). This step is deemed as the most important step of PS, differentiating it from a routine exercise, as outlined by Polya (Bodner & Herron, 2002).
Although OC is an information heavy course (Anim-Eduful & Adu-Gyamfi, 2022b), memorizing alone is neither a feasible nor an effective approach. At its core, PS yields outcome that reinforce, revise or add to the existing knowledge (Lyle & Robinson, 2001). This is crucial because new content builds upon a fundamental understanding of previously learned topics. If misconceptions persist throughout the course, learning becomes significantly more challenging (Anim-Eduful & Adu-Gyamfi, 2022a, 2023) and may result in reverting to memorization. However, this approach is inadequate given the substantial number of course objectives found in OC courses.
Understanding the internal and external factors that can limit students when attempting to solve problems is crucial. The “working memory overload hypothesis” proposed by Johnstone and El-Banna (1986) suggests that cognitive demand is a limiting factor in PS. This concept involves the process of assimilating auditory and visual information from daily life into both working and long-term memory (Baddeley, 2000). It includes demands stemming from outside factors in a student’s life, such as the workload for other courses, responsibilities to other institutions, physical and mental health, and socioeconomic status. This collective influence directly affects students’ attributes, including confidence, self-efficacy, anxiety, fatigue, and stress, all of which profoundly impact students’ motivation and PS abilities (Jonassen, 2010).
Moreover, spatial ability can significantly impact a student’s PS capacity. Spatial ability involves the capacity to conceptualize and visualize the movement of objects without a physical change. This correlation was identified through the Purdue visualization of rotations (ROT) test, a spatial ability assessment (Ekstrom et al., 1976). A study revealed a clear correlation between ROT test scores and performance on PS tasks in chemistry (Bodner & Guay, 1997). These tasks often require the use of cognitive restructuring and dis-embedding strategies, which are crucial aspects of PS.
Beyond these internal factors, difficulties in PS are deeply rooted in the history of chemistry. The historical development of chemistry is intertwined with the practices of scientific colonialism, stemming from periods of imperialism and the transatlantic slave trade (Hira, 2015). This practice was employed to justify white European dominance over other groups and, more significantly, to create an “empirical discourse, where whiteness becomes the arbiter of who is a valid observer and practitioner of science,” exploiting others and their power in the name of the greater good (Clark & Walsh, 2009). Consequently, science has been traditionally taught from a hetero-cis-normative, colonial, and predominantly white perspective. This is one reason why the science, technology, engineering, and mathematics fields have been historically dominated by white men; these disciplines are grounded in logic and reasoning, two essential elements of PS that have not been equally accessible to everyone.
This historical perspective, deeply ingrained in the system for centuries, poses a significant challenge when it comes to teaching chemistry to a diverse group of students. Professors may have a deep understanding of the course material, but they often struggle to connect with their audience. This issue is first evident in the language that is used. Instructors often assume that students are familiar with certain terminology while explaining concepts, which can be counterproductive (Vincent-Ruz, 2020). Attempting to explain the unknown using more unknown terms can leave students feeling discouraged and helpless. This is especially true for marginalized students, such as those for whom English is not their first language or students who lack a strong foundation in science. The terminology can create an uncomfortable learning environment, leading students to blame themselves and question their abilities when they do not grasp what is labeled as “basic language”. Due to time constraints and the structure of courses, students may fixate on this thought for the remainder of the class period, ultimately falling behind and feeling discouraged. Consequently, students may struggle to engage in CT within the course, not because of their intelligence or cognitive abilities, but due to their familiarity with material that has been influenced by inequitable social systems (Vincent-Ruz, 2020).
This uncomfortable learning environment is further exacerbated by the diverse backgrounds, cultures, and upbringings of many students. In various cultures, it is considered inappropriate to question individuals of higher “social status” or older age. This cultural norm can serve as an explanation as to why numerous students refrain from expressing their misunderstandings–they may simply not know how to do so. Additionally, it is challenging to articulate one’s misunderstanding about an abstract topic, as the confusion might not be well-defined. As a result, students often resort to asking general questions or requesting repetitions, which do not effectively address their specific confusion. Even when students manage to find the courage to ask questions, they may not receive adequate support from instructors, as many educators are well-versed in the material but may lack training in effective teaching methods (Bang et al., 2012). It is unrealistic to expect students to understand when the learning environment has been systematically structured in a way that perpetuates misunderstanding.
Perhaps another reason students struggle with PS is their difficulty in connecting OC with their everyday lives (Own et al., 2010). This issue significantly further contributes to and impacts the learning environment. Students often lack intrinsic motivation to engage with course material in a meaningful, long-term manner. This motivation deficit can be attributed, in part, to the presentation of information. Students frequently shape their study habits and interests based on what professors emphasize and discuss in class. In other words, when professors stress textbook readings and the memorization of facts, students tend to adopt a similar approach, focusing solely on achieving a letter grade. Thus, a student’s primary goal shifts from genuine learning to the mere acquisition of grades. This overemphasis and focus on memorization become problematic when students are tested on the material. Even though they may have identified all the essential pieces of information, they may not know how to use them or arrange them to construct a logical answer. In fact, when there is an overload of information, it prevents the brain from actually processing the information, thereby impeding PS (Wood, 2006). This further solidifies a student’s lack of motivation.
Although instructors often emphasize memorization and textbook readings subconsciously, it is important to recognize that these factors are direct contributors to students’ struggles in PS. Nevertheless, there are many strategies to help stimulate higher-order thinking. For example, showing mechanisms behind different reactions has been demonstrated to improve understanding and, consequently, PS (Flynn & Ogilvie, 2015). Mechanisms provide insight and help fill the gap in the “why” and “how” of reactions, facilitating PS. Understanding concepts such as electron flow, the presence of leaving groups, and acidic/basic conditions for use in mechanisms allows students to develop intuition and predictive capabilities for a wider range of problems. This approach is quite different from memorizing specific nucleophilic attacks or reagents, as mechanisms rely on understanding general trends, such as identifying electron-rich sources. In fact, many of these concepts are covered in detail in the general chemistry course, but professors often present them as new material in upper-level courses. Demonstrating various mechanisms enables students to identify trends between different reactions and further develop an understanding of the facts they have previously memorized. This approach allows the material learned in any given section to be integrated across different topics, fostering extensive and flexible knowledge rather than mere fact memorization for short-term use (Vermunt & Donche, 2017). Consequently, this method of expanding knowledge directly allows for more effective and vast PS skills (English & Gainsburg, 2016).
One effective method to foster PS skills is to externalize internal concepts and questions. This approach includes techniques such as problem manipulation, drawing diagrams, trial and error, and other visualization methods to gain a better understanding of a given problem. To implement this, students can annotate the problem, identifying aspects they recognize and those that require self-awareness. Among the strategies recommended by experts is “the whiteboard method,” where students use an empty canvas to freely externalize their thoughts associated with the problem, promoting agility in PS (Hmelo-Silver, 2004). This method allows students to express all their thoughts without the pressure of immediately settling on a final answer. After solving the problem, students can reflect on their thought process and approach patterns. To further promote this externalization method, students can observe various experts externalizing their thought processes when tackling problems. It is important to understand that instructors often write out PS steps and narrate the process rather than thoroughly explaining it. Since students tend to model their engagement with course material based on what they observe from professors, it is crucial for instructors to demonstrate effective strategies for PS (Hmelo-Silver, 2004).
This comes as a direct result of the discrepancy that exists between what instructors typically do when approaching a problem versus what they expect from students. Systematically speaking, lecture courses are structured with content experts who often explain problems as they perceive them, which is typically routine exercises (Bodner, 2003). Thus, when modeling a problem, essential PS aspects like manipulating questions are not explicitly shown to students. One of the most challenging aspects of PS is understanding the question itself. This misperception often leads students to believe that PS in OC is all intuition and that if the student looks at a problem and does not know what to do immediately, then the student will not thrive in the course. This perception undermines the need for students to manipulate problems to develop effective strategies. Consequently, many students rarely test their potential answers to determine their logical validity.
Another frequently overlooked element is the final step of Polya’s PS method–holistic problem evaluation. Many students fail to implement this step. While their answers may have the right components and reasoning, they may lack a logical order or may contain contradictory components. For instance, in mechanism questions, if a reaction takes place under acidic conditions, the presence of a base would neutralize the reaction. While this may seem intuitive to an instructor with extensive knowledge, it is essential to explicitly clarify such issues for students. Nevertheless, observing others working through problems can promote and expose students to different strategies for tackling them. With enough practice and understanding of the course material, students can identify PS trends and develop their unique PS methods.
Externalizing class material can also take the form of collaborative learning. While students asking questions is vital for learning, it is equally important for professors to encourage students to question (Hmelo-Silver & Barrows, 2008). The classroom setting must be welcoming to student engagement so that material can be exchanged between all parties. Each student provides a unique approach to material. From different memorization mnemonics to PS strategies, collaborative learning has been proven effective (Hmelo-Silver & Barrows, 2008). Students are more likely to share their insights in small group settings, where the stakes are lower. Peer-led team learning (PLTL) serves as an excellent outlet for this. PLTL involves a small group of students facilitated by another student who successfully completed the course and received training in learning theory. In these small settings, the peer leader works out problems and reinforces concepts in chemistry. This environment offers students an opportunity to work on problems both independently and collaboratively, something often limited in large lecture courses. PLTL has been associated with “improved student performance, retention, and learning experiences, and has also earned the interest of faculty, peer leaders, and participants themselves” (Stephenson et al., 2019). Events and experiences are often more memorable than facts, and this method turns OC into an experience rather than just a subject. Having a peer leader who completed the course is what makes this strategy particularly effective.
Studies have also shown an alternative method to a traditional lecture course known as problem-based learning (PBL) (Barrows, 2000; Torp & Sage, 2002). PBL is a “focused, experiential learning organized around the investigation, explanation, and resolution of meaningful problems” and directly helps students develop PS skills (Barrows, 2000; Torp & Sage, 2002). Misconceptions and deficiencies in the material are identified then followed up by self-directed learning. This strategy of learning makes PS transferable to other questions. In a study done on a group of medical students, those who were taught using PBL “more likely to produce accurate hypotheses and coherent explanations than students in the traditional curriculum” and “were more likely to use science concepts in their explanations” than their counterparts who were taught in a traditional curriculum (Hmelo-Silver, 2004). In fact, PBL produces effective PS and long-term success. In regards to clinical PS and clinical performance tests, PBL students performed better and were better able to deal with different problems regardless of their familiarity with the problem (Albanese & Mitchell, 1993; Norman et al., 1994; Vernon & Blake, 1993). As mentioned previously, a vital aspect of effective PS is noticing trends and building an extensive, flexible knowledge. PBL has indeed shown to promote this as beyond course success, it produces logical and effective professionals. In fact, learning effective PS skills benefits many students in the future. When employers look for new hires, they look for chemistry graduates with higher-order cognitive skills including CT, connection making, and analysis (St Clair-Thompson et al., 2012).
PBL method has been shown to benefit students across various science backgrounds. Students of varying majors who initially fell short of the national board of medical examiners part I benchmark were able to enhance their study habits through PBL and achieve academic success (Hmelo-Silver, 2004). For PBL to be effective, self-awareness and self-directed learning must be reinforced, where high-self regulated learners do not struggle with adapting to PBL (Robinson & Persky, 2020).
A significant aspect of effective PS relies on students’ understanding of their own needs and learning strategies. This includes the resources they use for learning and understanding. PBL students are more likely to create and discover resources on their own, while conventional students often rely on materials provided by faculty (Stefanou et al., 2013). There is often a gap between how instructors perceive students’ learning and how students actually learn. Materials selected by faculty often follow a “one size fits all” approach, which may not cater to each student’s unique learning style. It is not feasible to create resources that work for all students due to the myriad factors that influence learning. However, with proper awareness and support, students can develop their materials and strategies for approaching problems.
As demonstrated by past studies, effective PS skills are crucial for completing higher-order tasks. The development of these skills is necessary for success in OC. With this in mind, we predict that students with strong PS skills, such as annotation, creating independent study materials, applying conceptual frameworks, and CT, will excel in OC.
Guiding Research Questions
-
How do internal and external factors influence a student’s PS capacity in OC?
-
What does a student’s ability to articulate their misconceptions say about their PS capabilities?
METHODS
The purpose of this study was to examine the multifaceted aspects of PS within the realm of OC. The research sought to examine the various internal and external factors, as well as PS skills, that impact a student’s ability to succeed in OC. In order to gain further insight into these aspects, a questionnaire was generated and distributed. The survey was subsequently administered to the target demographic: students currently enrolled at the City College of New York (CCNY), both those presently taking OC courses and those with past experience in the subject. Data collection took place during the Spring and Fall semesters of 2022, with student participation being entirely voluntary and ensuring confidentiality. In total, 175 surveys were distributed, and 128 responses were received, providing valuable data on the subject. The target population for this study are students who have completed at least organic chemistry I course. As a result, we recruited students enrolled in organic chemistry II, organic chemistry I and organic chemistry II laboratories, and biochemistry courses.
The cross sectional survey included both Likert-type and open-ended questions to gather information about students’ experiences with PS in OC. To gain a deeper understanding of how students approach problems, short-answer, and multiple-choice questions were based on OC content. Students were encouraged to provide detailed responses in the manner they saw fit. In addition to analyzing students’ PS methods for specific questions, students were asked to explain their general expectations regarding PS. To understand any potential barriers that students might encounter, an open-ended question prompted students to explain both what limitations they faced and why these limitations existed. Likert scale questions were employed to further assess students’ confidence levels and beliefs regarding factors that impact their PS skills. The phrasing of these questions aimed to gain insight into the physical and cognitive processes students employ when solving problems in OC.
The administration of the survey and data gathering were approved by CCNY Internal Review Board. The survey findings were examined using a variety of visualization techniques, such as graphs to show students’ responses, histograms to display the percentage of correct answers for each multiple-choice question. Two specialists who reviewed the survey concurred that the questions accurately reflect the inquiry into students’ perceptions about PS ability and its relationship to learning and performance in OC. The reliability coefficient was calculated using the test-retest approach and was found to be 0.87. The Likert-type questions were subjected to a single factor ANOVA, which revealed p<.001 and p<0.05, which is strong evidence against the null hypothesis and demonstrates a strong association between the variables.
The Likert-type section had a five-point scale, including strongly disagree (1), disagree (2), neutral (3), agree (4), and strongly agree (5). Responses above 3.5 indicated a certain level of agreement with the statements, while responses between 2.5 and 3.5 signified neutrality, and responses under 2.5 indicated disagreement to some extent. In the next section of the survey, there was a single open-ended question that aimed to understand the role of annotation in PS. This was significant in gauging the extent to which students rely on annotation when faced with PS. For this open-ended question, a rubric was utilized to score the answers on a one to five scale. The analysis of the short-response data was conducted qualitatively, considering specified criteria, to ensure that students were able to express themselves accurately.
RESULTS & DISCUSSION
Table 1 employs a Likert-type questionnaire to examine students’ perspectives on PS in OC, revealing notable trends. Question 1 and question 10 indicate a positive inclination towards annotating problems (mean=4.08), emphasizing its value as an effective PS strategy. Questions 2, 3, 4, and 7 collectively stress the importance of strong foundational understanding (mean=3.95), highlighting the role of conceptual comprehension and quality teaching. In contrast, questions 5, 6, 8, and 9 underscore the significance of peer learning and external resources (mean=4.05), promoting collaborative learning and diverse educational tools as valuable assets for OC PS. These trends offer insights for educators and curriculum developers to enhance the overall learning experience of OC.
Table 1. Likert-type & one open-ended questions & average answer from respondents
|
In the bar graph above, Figure 1 demonstrates the analysis of students’ perceptions, which revealed several key challenges faced in learning OC. Approximately 29.2% of participants found memorizing all the rules and reactions to be troublesome. About 23.1% of students reported difficulty in understanding the underlying concepts, specifically the rules and reactions. Students also encountered difficulties in PS, with 10.8% struggling with carrying out mechanisms and 7.7% having issues with devising multistep synthesis. Furthermore, 12.3% of students found the overall workload of OC, both in terms of material and time, challenging, while 10.7% grappled with the extensive range of topics covered in the course. Additionally, 6.2% of participants experienced difficulties related to spatial ability, a critical skill in OC PS.
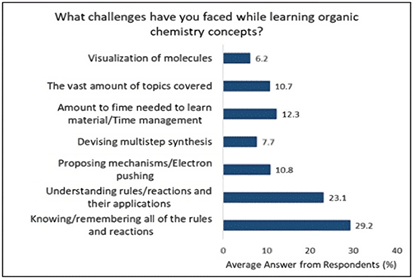
When tasked with identifying the weakest base, out of the 128 participants, 71 answered correctly, 44 answered incorrectly, and 13 did not provide an answer. Figure 2 presents the rationale of students based on their responses to the question about identifying the weakest base.
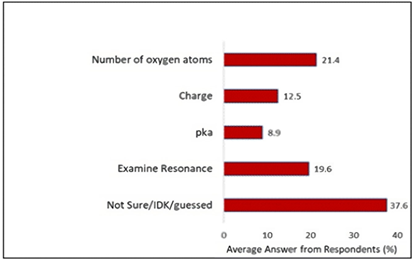
In Figure 2, students who expressed uncertainty and made conjectures (even if they were unable to pinpoint the correct compound) were included. Nearly 40.0% of the participants who answered incorrectly or could not answer at all were unable to articulate a clear thought process for addressing acid-base questions. Some merely demonstrated a superficial grasp of the material, basing their answers on factors such as charge or the number of oxygen atoms.
Aside from annotation, trial and error also emerged as a valuable PS approach. Many students reported in Table 1 that observing experts in class, recitations, or online videos helped them develop their own strategies. As students depend on these sources to model effective PS, it becomes imperative that techniques like trial and error are showcased (Hmelo-Silver, 2004). Failure to demonstrate the learning process, including potential mistakes, could potentially hinder the development of effective PS techniques and erode students’ confidence.
According to Table 1, students, on average, expressed neutrality in their confidence levels. This likely suggests that their hesitation to provide answers or reliance on guessing, without showing any attempts in both the synthesis and acid-base questions, may be rooted in the belief that a clear train of thought should mirror that of experts–instantaneous and flawless. When this expectation is not met, students may perceive it as a dead end, leading to premature surrender. Significantly, all students who cited “I do not know” as their rationale did not make any attempts. This data underscores how poor confidence can exacerbate the disconnect between students’ expectations and the reality of PS.
On the other hand, in Figure 3, those who answered correctly were able to specify the factors related to charge or atoms that made the third molecule the most basic. They could delineate how the location of the charge and the inductive effects of the double-bonded oxygens on both sides contributed to this determination. This understanding of the rules enabled these students to answer correctly and provide a reasonable justification. It is worth mentioning that both groups acknowledged the significance of resonance as a rationale. Specifically, 30.5% of those who answered correctly and 19.6% of those who answered incorrectly justified their responses by invoking resonance.
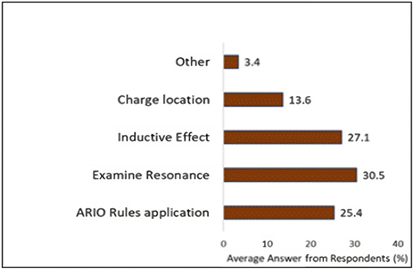
Following the presentation of the results, it is evident from Table 1 that students nearly unanimously agreed about the significance of understanding effective PS as seen by their agreement regarding question inquiry, modeling observation, and annotation. Unfortunately, when participants transitioned from this belief to actual PS performance, there was no unanimity. While some students adeptly tackle questions like acid-base problem, many did not. Discrepancy exposes the gap between students’ perceived strategies and their practical application. To delve deeper into this phenomenon and unearth potential areas for improvement, Likert scale and short response questions were instrumental in identifying prevailing trends among participants.
For instance, in Table 1, most students concurred on the significance of annotation, even expressing their likelihood of adopting this practice. Surprisingly, in the context of synthesis and acid-base questions, only a minority of students actually used annotation, despite many acknowledging its importance. Annotation serves as a method for students to strategize and explore PS avenues. Notably, those who employed annotation as a strategy successfully navigated the acid-base question. While only a subset of students drew resonance arrows for justification in Figure 3, those who did were able to answer correctly. Annotation empowered students not only to explain why their answer was correct but also to reinforce their understanding of why other choices were incorrect (Hmelo-Silver,2004). This ability aligns with the widely accepted PS model developed by Polya. It plays a crucial role in externalizing what is often a complex internal thought process, expanding students’ knowledge and cementing information into their PS toolkit (Bodner, 2003). Such students can minimize their reliance on memorization and instead focus on justifications when tackling OC problems (Hmelo-Silver, 2004).
Many students still gravitate towards memorization, even though they are aware that OC demands higher-order cognitive skills. Our data indicates that students expressed neutrality when asked about memorization while concurring on the importance of understanding, as evident in Table 1. However, Figure 1 reveals that students grappled more with memory than with understanding. Although memorization is essential for selecting the correct reagents and deducing the products, the volume of memorization could be significantly reduced if students could discern commonalities among the concepts (Barrows, 2000; Torp & Sage, 2002). This would facilitate effective, proactive memorization and cultivate an intuitive approach to a wide array of problems (Lyle & Robinson, 2001). Thus, students who struggle with memorization may also grapple with mastering the foundational concepts, eventually becoming overwhelmed as the course progresses.
This struggle is further exemplified in the justification for the acid-base answers. In Figure 2 and Figure 3, it is noteworthy that resonance was employed as a justification by both groups. Resonance, by definition, involves the delocalization of electrons to highlight various bonding structures within a molecule. It typically involves the presence of charges, electronegative atoms, and pi bonds (Wei & Chu, 2023).
While both groups recognized that the molecules could potentially exhibit resonance, based on established rules, only those who could successfully delocalize the electrons arrived at the correct answer. This underscores the notion that solely memorizing rules is ineffective unless students possess the requisite understanding to apply these rules effectively (Lyle & Robinson, 2001).
CONCLUSIONS
In the realm of OC, this study sheds light on the critical aspects of PS, providing a deep understanding of the challenges and complexities faced by the students. By meticulously examining both the internal and external factors, our research contributes significantly to the academic knowledge, offering valuable insights to the multi-faceted nature of PS in this demanding subject.
The crucial role of annotation as a powerful PS tool is underscored in our findings. While students acknowledge its significance, there exists a gap between this awareness and practical application. Effective problem-solvers demonstrate the ability to externalize their cognitive processes through annotation, reducing reliance on rote memorization and deepening their understanding of OC. Additionally, the study emphasizes the importance of trial and error and modeling as effective PS strategies, highlighting the need for transparent and authentic demonstrations of the learning process, both in the classroom and through online resources. This not only builds students’ confidence but also enhances their PS skills.
Furthermore, while memorization remains essential, coupling it with a comprehension of the subject can help alleviate the burden on memory and empower students to recognize patterns apply principles more flexibly and intuitively. In conclusion, this study provides a comprehensive understanding of the challenges encountered by students in OC, offering pedagogical implications applicable to diverse domains. Educators and students can leverage these insights to enhance PS strategies, bridging the gap between theory and practice and fostering more effective learning experiences in the complex domain of OC.
Author contributions: All authors have sufficiently contributed to the study and agreed with the results and conclusions.
Funding: No funding source is reported for this study.
Ethical statement: The authors stated that the study was approved by Human Research Protection Program on 19 April 2022with approval number 2022-0256-CCNY. Written informed consents were obtained from the participants.
Declaration of interest: No conflict of interest is declared by the authors.
Data sharing statement: Data supporting the findings and conclusions are available upon request from the corresponding author.
References
- Albanese, M. A., & Mitchell, S. (1993). Problem-based learning: A review of literature on its outcomes and implementation issues. Academic Medicine, 68, 52-81. https://doi.org/10.1097/00001888-199301000-00012
- Anim-Eduful, B., & Adu-Gyamfi, K. (2022a). Chemistry students’ conceptual understanding of organic qualitative analysis. Pedagogical Research, 7(4), em0132. https://doi.org/10.29333/pr/12307
- Anim-Eduful, B., & Adu-Gyamfi, K. (2022b). Factors influencing high school chemistry teachers’ and students’ teaching and learning of organic qualitative analysis: A qualitative study. European Journal of Education Studies, 9(7), 194-219. https://doi.org/10.46827/ejes.v9i7.4378
- Anim-Eduful, B., & Adu-Gyamfi, K. (2023). Nature of senior high school chemistry students’ alternative conceptions in organic qualitative analysis. Aquademia, 7(2), ep23007. https://doi.org/10.29333/aquademia/13711
- Baddeley A. D. (2000). The episodic buffer: A new component of working memory? Trends in Cognitive Science, 11, 417-423. https://doi.org/10.1016/s1364-6613(00)01538-2
- Bang, M., Warren, B., Rosebery, A. S., & Medin, D. (2012). Desettling expectations in science education. Human Development, 55, 302-318. https://doi.org/10.1159/000345322
- Barrows, H. S. (2000). Problem-based learning applied to medical education. Southern Illinois University Press.
- Bodner, G. M. (2003). Problem solving: The difference between what we do and what we tell students to do. University Chemistry Education, 7(7), 37-45.
- Bodner, G. M., & Guay, R. B. (1997). The Purdue visualization of rotations test. The Chemical Educator, 2(4). https://doi.org/10.1007/s00897970138a
- Bodner, G. M., & Herron, J. D. (2002). Problem-solving in chemistry. In J. K. Gilbert, O. Jong, R. Justi, D. F. Treagust, & J. H. Driel (Eds.), Chemical education: Towards research-based practice (pp. 235-266). Springer. https://doi.org/10.1007/0-306-47977-X_11
- Bodner, G. M., & McMillen, T. L. (1986). Cognitive restructuring as an early stage in problem-solving. Journal of Research in Science Teaching, 23(8), 727-737. https://doi.org/10.1002/tea.3660230807
- Clarke, S., & Walsh, A. (2009). Scientific imperialism and the proper relations between the sciences. International Studies in the Philosophy of Science, 23, 195-207. https://doi.org/10.1080/02698590903007170
- Ekstrom, R. B., French, J. W., & Harman, H. H. (1976). Manual for kit of factor referenced cognitive tests. Educational Testing Service.
- English, L. D., & Gainsburg, J. (2016). Problem solving in a 21st-century mathematics curriculum. In L. D. English, & D. Kirshner (Eds.), Handbook of international research in mathematics education (pp. 313-335). Routledge.
- Flynn, A. B., & Ogilvie, W. W. (2015). Mechanisms before reactions: A mechanistic approach to the organic chemistry curriculum based on patterns of electron flow. Journal of Chemical Education, 92(5), 803-810. https://doi.org/10.1021/ed500284d
- Hira, S. (2015). Scientific colonialism: The eurocentric approach to colonialism eurocentrism, racism and knowledge: Debates on history and power in Europe and the Americas. In M. Araújo, & S. Rodríguez Maeso (Eds.), Eurocentrism, racism and knowledge (pp. 136-153). Springer. https://doi.org/10.1057/9781137292896_8
- Hmelo-Silver, C. E. (2004). Problem-based learning: What and how do students learn? Educational Psychology Review, 16(3), 235-266. https://doi.org/10.1023/B:EDPR.0000034022.16470.f3
- Hmelo-Silver, C. E., & Barrows, H. S. (2008). Facilitating collaborative ways of knowing. Cognition and Instruction, 26, 48-94. https://doi.org/10.1080/07370000701798495
- Johnstone, A. H. & El-Banna, H. (1986), Capacities, demands, and processes- a predictive model for science education. Education in Chemistry, 23, 80-84.
- Jonassen, D. H. (2010). Learning to solve problems: A handbook for designing problem-solving learning environments. Routledge. https://doi.org/10.4324/9780203847527
- Lyle, K. S., & Robinson, W. R. (2001). Teaching science problem solving: An overview of experimental work, Journal of Chemical Education, 78, 1162-1163. https://doi.org/10.1021/ed078p1162
- Norman, G. R., Trott, A. D., Brooks, L. R., & Smith, E. K. (1994). Cognitive differences in clinical reasoning related to postgraduate training. Teaching and Learning in Medicine, 6, 114-120. https://doi.org/10.1080/10401339409539657
- Own, Z. Y., Chen, D. U., & Chiang, H. R. (2010). A study on the effect of using problem-based learning in organic chemistry for web-based learning. International Journal of Instructional Media, 37(4), 417-431.
- Robinson, J. D., & Persky, A. M. (2020). Developing self-directed learners. American Journal of Pharmaceutical Education, 84(3), 847512. https://doi.org/10.5688/ajpe847512
- St Clair-Thompson, H., Overton, T., & Bugler, M. (2012). Mental capacity and working memory in chemistry: Algorithmic versus open-ended problem solving. Chemistry Education Research and Practice, 13(4), 484-489. https://doi.org/10.1039/C2RP20084H
- Stefanou, C., Stolk, J. D., Prince, M., Chen, J. C., & Lord, S. M. (2013). Self-regulation and autonomy in problem- and project-based learning environments. Active Learning in Higher Education, 14(2), 109-122. https://doi.org/10.1177/1469787413481132
- Stephenson, N. S., Miller, I. R., & Sadler-McKnight, N. P. (2019). Impact of peer-led team learning and the science writing and workshop template on the critical thinking skills of first-year chemistry students. Journal of Chemical Education, 96(5), 841-849. https://doi.org/10.1021/acs.jchemed.8b00836
- Torp, L., & Sage, S. (2002). Problems as possibilities: Problem-based learning for K-12 education. ASCD.
- Vermunt, J. D., Donche, V. A (2017). Learning patterns perspective on student learning in higher education: State of the art and moving forward. Educational Psychology Review, 29, 269-299. https://doi.org/10.1007/s10648-017-9414-6
- Vernon, D. T., & Blake, R. L. (1993). Does problem-based learning work?: A meta-analysis of evaluative research. Academic Medicine, 68, 550-563. https://doi.org/10.1097/00001888-199307000-00015
- Vincent-Ruz, P. (2020). What does it mean to think like a chemist? In K. Y. Neiles, P. S. Mertz, & J. Fair (Eds.), Integrating professional skills into undergraduate chemistry curricula (pp. 57-79). American Chemical Society. https://doi.org/10.1021/bk-2020-1365.ch005
- Wei, S., & Chu, L. (2023). Resonance. Chemistry LibreTexts. https://chem.libretexts.org/Bookshelves/Physical_and_Theoretical_Chemistry_Textbook_Maps/Supplemental_Modules_(Physical_and_Theoretical_Chemistry)/Chemical_Bonding/Valence_Bond_Theory/Resonance
- Wood, C. (2006). The development of creative problem solving in chemistry. Chemistry Education Research and Practice, 7(2), 96-113. https://doi.org/10.1039/B6RP90003H
- Zoller, U. (1993). Lecture and learning: Are they compatible? Maybe for LOCS: Unlikely for HOCS. Journal of Chemical Education, 70, 195-197. https://doi.org/10.1021/ed070p195
- Zoller, U., & Pushkin, D. (2007). Matching higher-order cognitive skills (HOCS) promotion goals with problem-based laboratory practice in a freshman organic chemistry course. Chemistry Education Research and Practice, 8(2), 153-171. https://doi.org/10.1039/B6RP90028C
How to cite this article
APA
Salame, I. I., Abdul Satter, S., & Warda, F. (2024). Examining students’ problem-solving approaches in organic chemistry and its impact on performance and learning. Interdisciplinary Journal of Environmental and Science Education, 20(2), e2407. https://doi.org/10.29333/ijese/14421
Vancouver
Salame II, Abdul Satter S, Warda F. Examining students’ problem-solving approaches in organic chemistry and its impact on performance and learning. INTERDISCIP J ENV SCI ED. 2024;20(2):e2407. https://doi.org/10.29333/ijese/14421
AMA
Salame II, Abdul Satter S, Warda F. Examining students’ problem-solving approaches in organic chemistry and its impact on performance and learning. INTERDISCIP J ENV SCI ED. 2024;20(2), e2407. https://doi.org/10.29333/ijese/14421
Chicago
Salame, Issa I., Serina Abdul Satter, and Fatima Warda. "Examining students’ problem-solving approaches in organic chemistry and its impact on performance and learning". Interdisciplinary Journal of Environmental and Science Education 2024 20 no. 2 (2024): e2407. https://doi.org/10.29333/ijese/14421
Harvard
Salame, I. I., Abdul Satter, S., and Warda, F. (2024). Examining students’ problem-solving approaches in organic chemistry and its impact on performance and learning. Interdisciplinary Journal of Environmental and Science Education, 20(2), e2407. https://doi.org/10.29333/ijese/14421
MLA
Salame, Issa I. et al. "Examining students’ problem-solving approaches in organic chemistry and its impact on performance and learning". Interdisciplinary Journal of Environmental and Science Education, vol. 20, no. 2, 2024, e2407. https://doi.org/10.29333/ijese/14421